Ultimet Biopsy Analyser
Get the readout in 60 minutes
Comprehensive analysis & conclusion for your patient
Benefit from parallelized & independent analysis
Connect UltiMet to your patient record to enrich your knowledge
UltiMet technology
This UltiMet technology is an outstanding platform that gives access to rapid, automated, and integrative conclusions for cancer diagnosis and therapeutic guidance.
The technology is based on cutting-edge in-line and continuous 3D single-cell tomography. Thanks to a high throughput optofluidics-based super-resolved analysis, UltiMet scans single cells’ nuclei, analyses images in real time, and detects cancer-related epigenetic biomarkers.
Our IA-based algorithm further classifies the tumor according to heterochromatin biomarkers and supports clinicians in diagnosing and treating patients who have cancer.
An integrative technology for biopsy analysis
A typical hospital journey for cancer diagnosis or therapeutic guidance includes the following steps:
- Patient engagement through a consultation with a clinician to determine which tests should be performed.
- Patient imaging and/or laboratory tests and /or biopsy.
- Genetic testing and/or imaging of the biopsy.
- Diagnosis or treatment plan performed by a board of oncologists, pathologists, histologists, and molecular biologists.
- Patient information and treatment.
UltiMet allows the automation and consolidation of the biopsy analysis from steps 2 to 4. It relies on a single-cell and profound analysis of the tumor epigenome.
Load your biopsies and get a deep analysis at the molecular level to quickly characterize the pathology and provide your patients with the best therapy.
Do you have any questions about this ProChip project or its applications? Visit the Prochip project page or give us your input.
Cancer Diagnosis
Following a clinical recommendation for diagnostic testing, the exact diagnostic testing method must be specified. Tests for diagnoses and therapeutic guidance vary widely in the information they provide, and often more than one will be requested with the aim of providing clinicians with more comprehensive information.
The use epigenetic cancer markers also holds potential for utilizing large data sets and sophisticated data analysis to create more standardized and automated conclusions from cancer tests analyses.
UltiMet is the answer to the precise and rapid diagnosis of cancer types and stages.
Therapeutic Guidance
Clinicians rely on the expert analysis of pathologists to make a diagnostic or therapeutic decision. Consultation with a pathologists is followed by communication of the clinical conclusion to the patient.
Due to the (ever increasing) complexity of cancer testing a clinical interpretation is rarely trivial. Pathology reports output from cancer testing can contain multiple types of information – such as data on histology, immunohistochemistry, genomic DNA and mRNA expression – meaning test interpretation required strong knowledge and experience, rarely producing discrete yes-no results.
UltiMet is the perfect solution to deciphering treatment decisions and guiding clinicians to optimise precision and personalized therapies.
What is the ultimet and prochip European projects?
The goal of this UltiMet Prototype show-off is to assess scientists’ and doctors’ interest in cancer-related applications by using a set of microfluidic products combined with early-stage technologies and new microfluidic designs.
This is a “to become” technology. Your feedback will help us further push its development for these specific applications.
For more information, refer to the H2020 project page.
Current limitations of cancer diagnosis, cancer treatment and therapeutic guidance
A brief history of cancer therapies
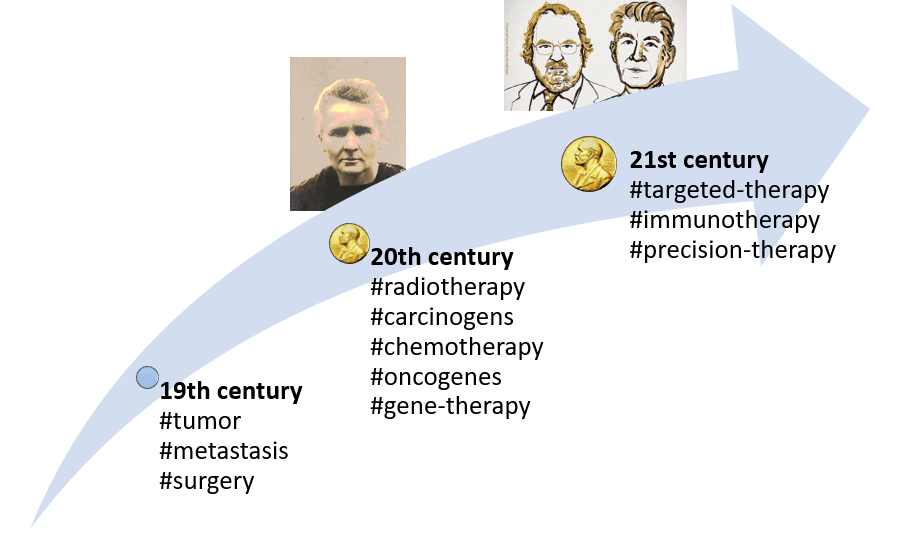
19th century
- 1829: Description of the invasion of the bloodstream by cancer cells: metastasis term coined (J. Recamier).
- 1845: First description of leukemia (J.H. Bennett).
- 1846: Anesthesia became available, allowing cancer surgery to spread widely.
- 1886: Hereditary basis for cancer first suggested (H. de Gouvea).
- The invention and use of the modern microscope helped identify cancer cells.
20th century
- Characterization of carcinogens (both artificial and natural).
- 1911: The second Nobel Prize was awarded to Marie Curie, which gave rise to radiotherapy as a critical cancer treatment.
- 1930: First statistical evidence for a connection between smoking and cancer.
- Chemotherapy (S. Farber, G. Elion) and hormone therapy (C. B. Huggins) have become golden cancer therapies.
- 1970: Childhood leukemia became one of the first cancers that a combination of drugs could cure.
- 1970s: Discovery of the first cancer gene (the oncogene).
- 1989: first approved gene therapy.
21st century
- 2000: The entire human genome is mapped.
- 2000s: After first targeting growth signal inhibitors, targeted therapies become potent tools for cancer treatment.
- 2018: Nobel Prize awarded to J.P. Allison and T. Honjo for their discovery of cancer therapy by inhibiting negative immune regulation, which gave rise to immunotherapies, towards precision therapies using expression profiling and proteomics.
Introduction to cancer: facts and figures
According to the NIH Cancer Institute, in 2018, an estimated 1,735,350 new cancer cases were diagnosed, and 609,640 people died from cancer in the United States [1]. In Europe, the World Health Organization (WHO) estimates over 3.7 million new cases and over 1.9 million deaths yearly [2].
At the European level, cancers represent 20% of deaths each year, with lung, breast, stomach, liver, colon, and breast cancer at the top of the list. Unsurprisingly, tobacco consumption is the most critical risk factor for cancer (together with alcohol, it accounts for 40% of cancers), while unhealthy lifestyles (poor diet, obesity…) account for 20% of cancers [2].
“Cancer represents 20% of deaths in Europe – this represents 1.9 million people dying every year” WHO/Europe.
Cancer diagnosis: well-established analyses at the cellular and tissue levels
When dealing with cancer treatment, the so-called “cancer continuum” must be taken into account. It includes prevention, screening, diagnosis, treatment, survivorship, and end-of-life. The PROCHIP H2020-FETOPEN project aims to develop a new approach to support medical decision-makers in the diagnosis and treatment steps.
Though it may appear to be trivial, cancer management starts with obtaining a valid diagnosis. The Cancer Atlas from the World Health Organization (WHO/Europe) highlights the lack of imaging and pathologists to achieve high-quality care [3].
Limitation #1: the lack of pathologists to provide a valid diagnosis
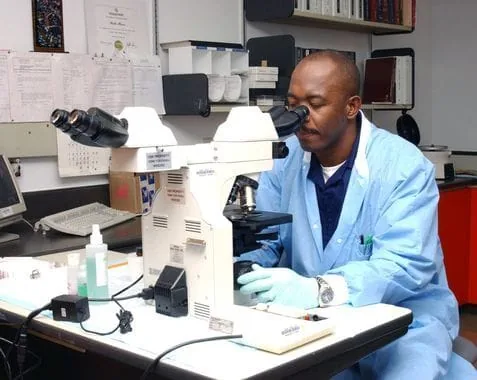
A series of elements are used to determine a treatment option or therapeutic guidance according to the developmental stage of a given cancer [1]:
- The tumor grade:
Tumor grade is determined by looking at cancer cells under a microscope. This is a physiological observation of the tissue structure. The less structured the tissue (or biopsy) and the less differentiated the cells, the more likely the tumor will be suspected to be malignant. Indeed, tumor cells grow and spread faster than usual or “well-differentiated” cells.
- The cancer stage: the TNM system
The cancer stage is often determined using the TNM system. The T (Tumor) refers to the size and extent of the main tumor, or so-called “primary tumor.” The N (Node) refers to the number of nearby lymph nodes with cancer. The M (Metastasis) refers to whether the cancer has metastasized, which means that it has spread from the primary tumor to other parts of the body.
- The patient: more case-to-case criteria such as the patient’s age and global healthiness.
Finally, each type of cancer may have specific elements affecting the classification.
Cancer treatment: how cancers are managed and treated?
Once a diagnosis has been properly done, the first line of treatment in 80% of early-stage cancer patients is surgery. Again, the WHO/Europe outlines the worldwide geographic inequity of available specialized surgeons.
Limitation #2: the lack of specialized surgeons to provide a first and crucial line of treatment to 80% of early-stage cancers
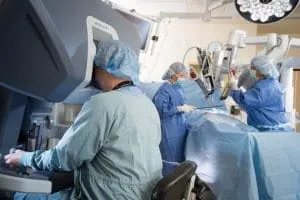
The second type of cancer treatment is radiotherapy. It is chosen for about 60% of cancers mainly for three reasons: as a palliative treatment, before surgery to shrink the tumor or after surgery to kill remaining cancer cells.
Limitation #3: the lack of available radiotherapy machines
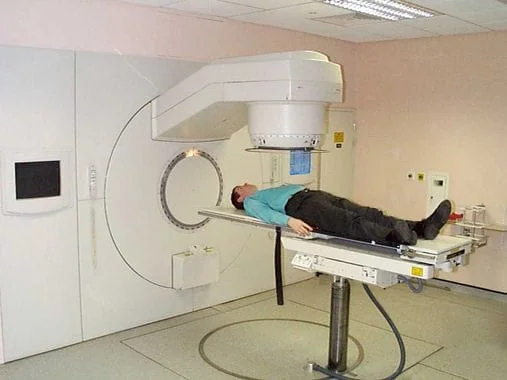
The third primary type of cancer therapy is chemotherapy. It has changed from systemic approaches to more personalized ones targeting receptor status, RNA expression, underlying DNA mutations, tumor environment, and immunologic responses over time.
Limitation #4: access to expensive drugs for chemotherapy treatment
It is essential to mention that despite the positive and crucial results obtained by standard approaches, a type of cells called “cancer stem cells” have been recently shown to be resistant to radiotherapy, chemotherapy, or immunotherapy, leading to mutated-resistant cells over time to the several treatment lines [5].
Cancer stem cells (CSCs) are self-renewable, immortal, and rare cells, which can lead to many cell types building a tumor.
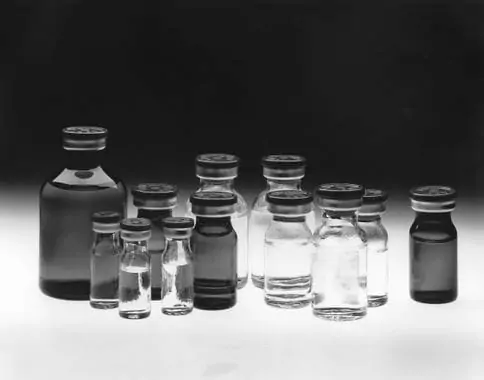
Limitation #5: cancer cells resistance to treatments, promoting mutated-resistant cancer cell populations
Another example of promoted resistance is the ability of tumors to develop therapeutic resistance by losing the target receptor in the context of CAR-T cell therapies and targeted immunotherapies.
One could then consider that some therapeutic guidelines open the road to the later apparition of resistance to targeted cancer treatments. The high division rate of cancer cells within tumors goes along with a high mutational rate.
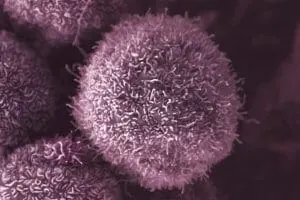
The probability of getting resistant cells is consequently high. These mutated CSCs will grow and divide into a resistant population of cells, resistant tissues, and potentially resistant circulating tumor cells (CTCs).
Exploring the possibilities to sample, observe, and analyze the cancer cells, considering their inherent variability within a tumor, is both a complex and necessary challenge that must be overcome to support medical decision-making and therapeutic guidance.
Focus on cancer stem cells (CSCS) and tumor heterogeneity to understand the importance of the tumor microenvironment.
“Cancer stem cells (CSCs) have been defined as cells with the capability of self-renewing, generating a heterogeneous population within the tumor” [6]. The phenomenon behind tumor growth is called “phenotypic switching.” It describes the ability of cancer cells to revert into CSCs and CSCs to be at the apex of a tree-type differentiating process leading to various types of cancer cells forming the tumor.
Identified regulators of this phenotypic switch between cancer stem cells and cancer cells (CCs) are micro-environmental factors.
This dynamic model, relying on the equilibrium between CSCs and CCs and the cell-cell interactions (or at least crosstalk) through micro-environmental factors, recently led researchers to revise the classical stochastic tumor development leading to metastasis.
Evidence that metastasis does not emerge from a single-cell type tumor mass detachment but more likely from a cluster of different CCs promotes a more plastic model for tumorigenesis where original CSCs play a determinant role [6].
Limitation #6: tumor heterogenicity and plasticity
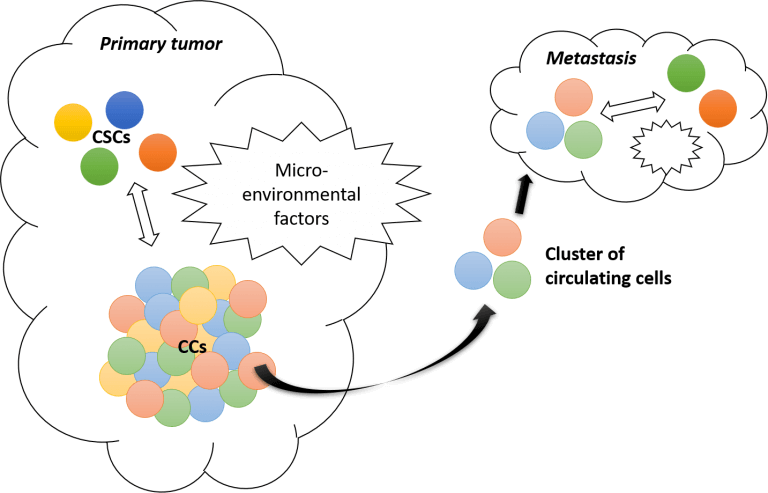
The phenotypic switching model points out the importance of tightly characterizing the heterogeneity of tumors at the single-cell level.
In light of these findings, metastasis could be due to one of these CCs with a hybrid phenotype that can easily migrate and switch in the right environment. According to this complex landscape, Wicha’s group showed that CSCs maintain plasticity to transition between mesenchymal-like and epithelial-like states in response to the tumor microenvironment [7].
There are also consequences for developing new therapeutic strategies based on the possibility of targeting essential mechanisms controlling the epithelial-mesenchymal transition (EMT) through the microenvironment. A mesenchymal–epithelial transition (MET) is a reversible biological process that involves the transition from motile, multipolar, or spindle-shaped mesenchymal cells to planar arrays of polarized cells called epithelia.
MET is the reverse process of EMT. “A characteristic feature of EMT landscape is loss of E-cadherin, causing adherens junction breakdown, which circumvents anoikis, promoting metastasis and chemoresistance” [4].
Limitation #7: the complexity of the scientific data to generate clear therapeutic guidelines
Conclusion to current limitations of cancer diagnosis
Limitations 1 to 4 primarily deal with demographics or access to specialized medical resources:
- #1 – the lack of pathologists to provide a valid diagnosis.
- #2 – the lack of specialized surgeons to provide a first and crucial line of treatment for 80% of early-stage cancers.
- #3 – the lack of available radiotherapy machines.
- #4 – access to expensive drugs for chemotherapy treatment.
However, limitations 5 to 7 reveal a crucial need for more precise cellular and molecular analyses:
- #5 – cancer cells’ resistance to treatments, promoting mutated-resistant cancer cell populations.
- #6 – tumor heterogeneity.
The latest limitation (#7) traduces the past decade’s tremendous scientific discoveries, new technical developments, and consequent data generation.
Biologists and cancerologists are facing a new challenge with the ongoing algorithmic revolution. While access to quantitative and qualitative datasets is right on our doorstep, efforts are now needed to homogenize datasets without standards for imaging, sequencing, phenotyping, etc.
Data analysis and the development of intelligent algorithms—such as artificial intelligence-based ones—are needed to help create new therapeutic guidelines that perform better than existing ones.
As the WHO/Europe organization recommended, “Research in high-income countries should also focus on implementation research as well as biological markers and precision medicine.” Hence, scientific advances and policy recommendations suggest that research in oncology should become increasingly precise and based on a tailored analysis of the tumor and its environment. Finding relevant biomarkers or new approaches is crucial in addressing this critical and ongoing “precision treatment” challenge.
The H2020-FETOPEN PROCHIP project aims to prototype such a solution thanks to advanced cancer cell sampling and cutting-edge imaging techniques. To deal with tumor heterogeneity, a two-level and parallel analysis will be required: first, this technology should decipher the spatial heterogeneity of tumors induced by the population of CCs coming from genetically distinct CSCs, and second, it should be able to get back to the cell level to identify precise mutations. A system for the high-throughput single-cell tracking of cells will be required to generate such a dataset.
References
1. E. Cazaly, J. Saad, W. Wang, C. Heckman, M. Ollikainen, and J. Tang, “Making sense of the epigenome using data integration approaches,” Front. Pharmacol., vol. 10, no. FEB, pp. 1–15, 2019.
2. F. Valdes-Mora et al., “Single-cell transcriptomics in cancer immunobiology: The future of precision oncology,” Front. Immunol., vol. 9, no. NOV, 2018.
3. H. A. Wani, S. A. Bhat, S. Majid, and S. Rashid, “Diagnostic utility of epigenetics in breast cancer – A review,” Cancer Treat. Res. Commun., vol. 19, p. 100125, 2019.
4. R. Siegel, C. DeSantis, and A. Jemal, “Colorectal cancer statistics, 2014,” CA. Cancer J. Clin., vol. 64, no. 2, pp. 104–117, Mar. 2014.
5. C. C. Wong, W. Li, B. Chan, and J. Yu, “Epigenomic biomarkers for prognostication and diagnosis of gastrointestinal cancers,” Semin. Cancer Biol., vol. 55, no. April, pp. 90–105, 2019.
6. S. Dedeurwaerder and F. Fuks, “DNA methylation markers for breast cancer prognosis,” Oncoimmunology, vol. 1, no. 6, pp. 962–964, Sep. 2012.
7. T. Mikeska and J. Craig, “DNA Methylation Biomarkers: Cancer and Beyond,” Genes (Basel)., vol. 5, no. 3, pp. 821–864, Sep. 2014.
8. L. Liu et al., “Targeted methylation sequencing of plasma cell-free DNA for cancer detection and classification,” Ann. Oncol., vol. 29, no. 6, pp. 1445–1453, Jun. 2018.