Droplet encapsulation for biological applications: a review
Published on July 23rd 2020
Droplet encapsulation creates microreactors to explore heterogeneity in biology
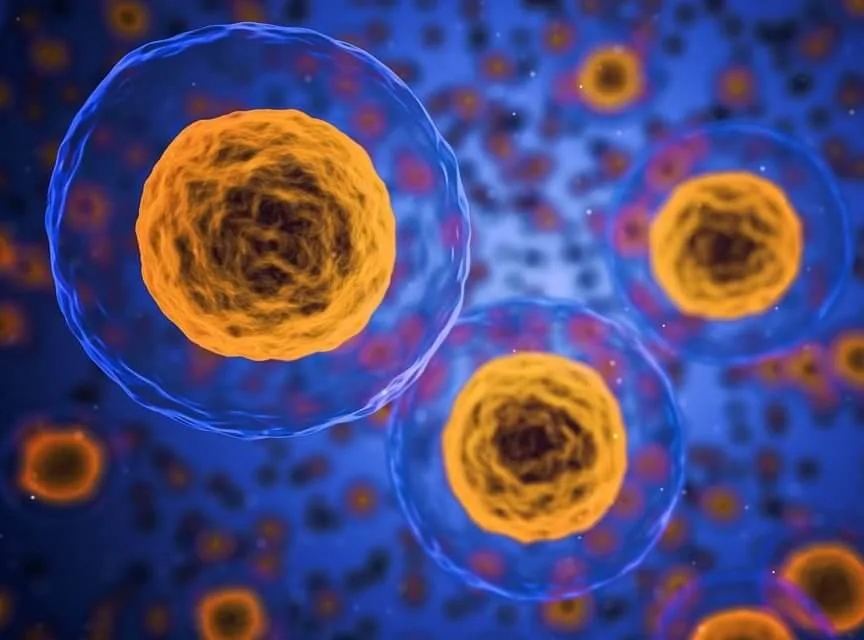
As biologists dive deep into the intricacies of cell and molecular biology, it becomes ever more apparent that many heterogeneities were being overlooked in traditional bulk analysis and experiments.
Exploring these subtleties without losing the speed and accuracy of conventional protocols is becoming a perfect application for microfluidics, especially encapsulation in droplets [1].
Droplet-based microfluidics are micrometer-sized droplet emulsions created in a microfluidic device. Using droplets in research is no novelty, but miniaturizing them to the microscale offers excellent advantages due to their larger surface-to-volume ratio.

This review will focus on the droplet encapsulation of samples and the potential applications of these microreactors in biology. You can check out our review for more information on droplet-based microfluidics.
Most used droplet encapsulation techniques
Droplet encapsulation techniques vary according to several factors, for example, the type and number of samples needed inside a single droplet and the desired order and time of mixing for chemical reactions. The most common form of encapsulation is to dilute the sample in the solution that will become the dispersed phase, in other words, the solution that will be turned into droplets [4].
Encapsulation during droplet formation
In single-cell droplet encapsulation, diluting the cells into the droplets’ dispersed phase before droplet formation is the most used method. The basic principle is to cut the cell suspension enough so that two cells will unlikely be inside the same droplet.
This approach is simple but wasteful, as it generates a lot of empty droplets since the probability of having one cell inside one droplet is relatively low: 36,8%. If there is a need to encapsulate two distinct cell types in the same droplet, or a cell and a bead, the probability of having one droplet with only one of each entity falls to 13,5%. It generates even more waste due to the higher dilution of the dispersed phase [1].
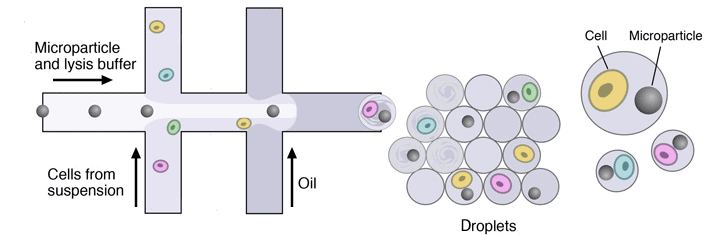
As mentioned in the two-cell example above, it is possible to have two different sample inlets as dispersed phases in a co-flow design, which will mix once they become a droplet [5].
It is also possible to merge two droplets that contain different reagents through electro-coalescence [6], which destabilizes the surface tension of surfactants so the two droplets can coalesce when in contact, or acoustic tweezer, which holds a droplet in place so flowing droplets can collide and merge into it [7].
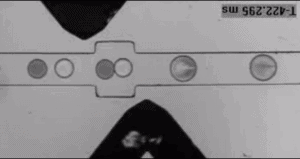
Encapsulation after droplet formation
Lastly, it is possible to inject reagents into existing droplets, giving more manipulation flexibility after droplet formation. There are two possibilities: plug-based and picoinjection. The plug-based microfluidic design uses a channel perpendicular to the flow of droplets to inject the new reagent via an injection droplet into the already-formed droplets [8]. And the picoinjection follows a similar procedure but uses an actuated electric field to have a sub-picoliter control of the injection [9].
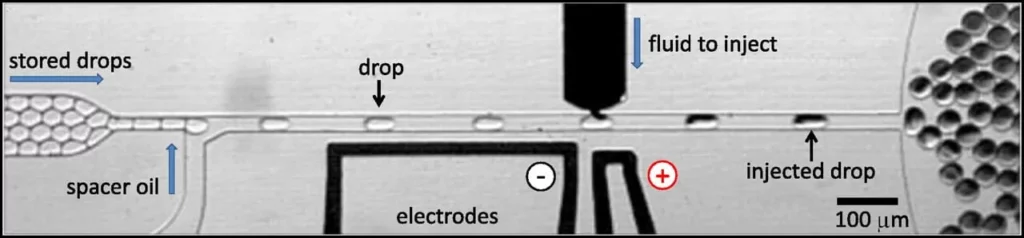
Droplet encapsulation as a tool for biological applications
High-throughput screening based on droplet encapsulation
High-throughput screening is a method of analyzing large libraries of compounds in a short time frame, for example, between 104 and 105 daily tests [11]. It has been widely used in drug discovery, toxicity, and antibody affinity assays with robotics and well plates.
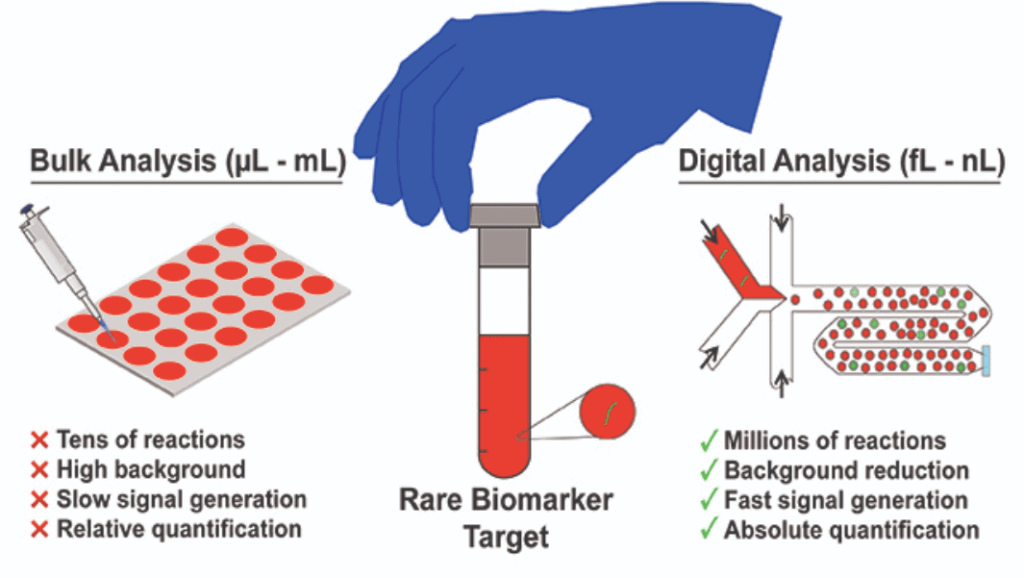
Droplet encapsulation is particularly well-suited to improve the outcomes of high-throughput screening because it allows for the generation of highly monodisperse droplets, which provides homogeneous reaction conditions. These reactions happen in the nL- and pL-range, reducing reagent consumption, and the droplets can be created with frequencies up to 10 kHz, allowing high-throughput screens of up to 100 million reaction conditions per day [12].
Some applications of droplet-based high-throughput screening are outlined in the sections below.
Single-cell analysis is optimized in droplet-based microfluidics
The advent of droplet-based microfluidics and droplet encapsulation allows biologists to screen for heterogeneity in cell populations through single-cell proteomics and genomics.
In single-cell proteomics, cells are confined in a minimal volume, which makes most biomarkers almost readily detectable. Either by cell secretion or cell lyses within the droplet, the detection of molecules of interest is usually done by fluorescence-labelled antibodies, providing a “0 or 1”-like result, which gives the name of digital microfluidics for this kind of analysis. There is a significant interest in the field to make the results quantifiable, opening even more possibilities for using droplets [1].
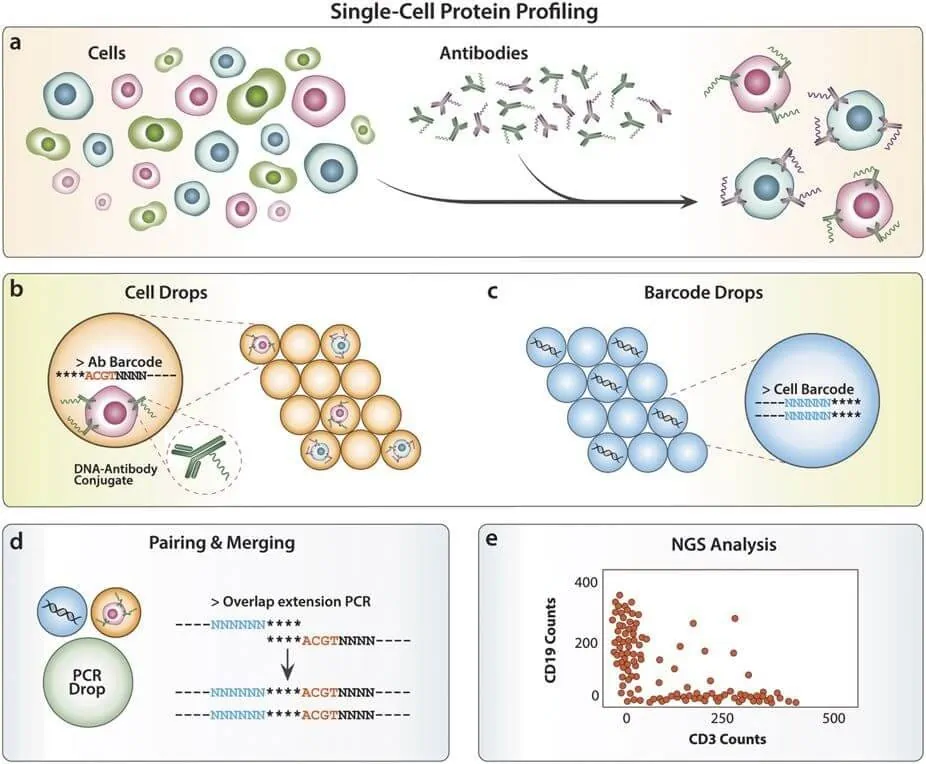
Single-cell genomics allows for DNA targeting by single-cell encapsulation with primer-functionalized beads. Therefore, it is possible to map the genomes of entire cell subpopulations with droplets. In this line, there is a commonly used sequencing protocol for RNA called Drop-seq, one of the few applications with commercial exploitation in this field to date [14].
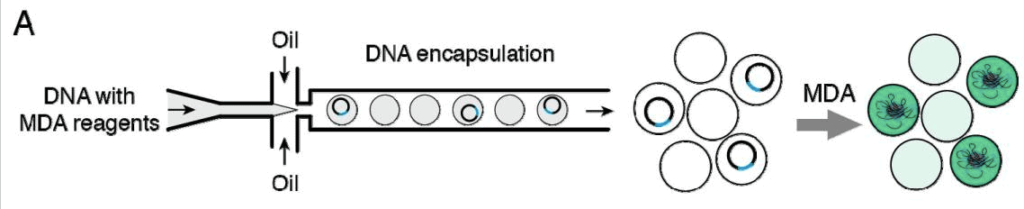
Droplet encapsulation enables ultrahigh-throughput in directed evolution
Directed evolution is a process that confines and speeds up the evolution of proteins by mimicking natural selection in vitro. It has been mainly applied to select and fine-tune interesting enzymes for industrial use.
The miniaturization, improved control and homogeneity, and sorting capabilities of droplet-based microfluidics allow researchers to develop ultrahigh-throughput directed evolution (over 106 compounds per day). These systems enabled the screening of 108 proteins, which exceeds the capabilities of standard approaches. For example, in a conventional plate assay, this experiment would take two weeks to screen only 2000 proteins [11].
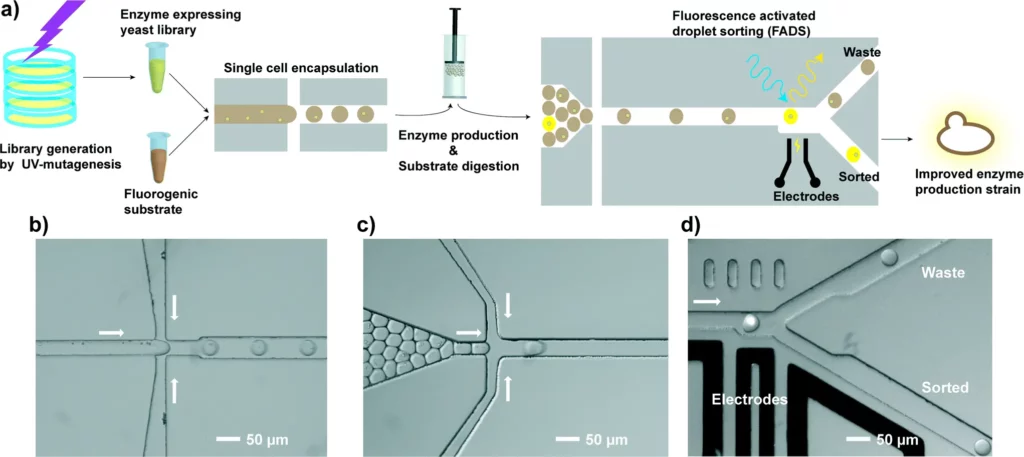
Droplet-based drug delivery systems
Besides being microreactors, droplets can be transformed into drug delivery systems (DDS). The droplet encapsulation methodology is similar to the ones described above, with the drug being diluted in the dispersed phase and its concentration being controlled by the flow rate.
However, depending on the administration route of the medication, the droplets might need to be turned into microparticles/nanoparticles through a solidification step. Also, some droplet-based DDS might have their solutions functionalized to respond to a specific pH or temperature, acting as controlled delivery systems [16].
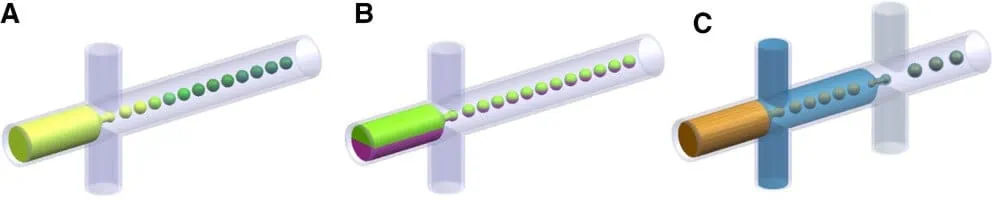
Droplets can be templates for artificial cells
Droplet compartmentalization can be used as a template for artificial cells. Depending on the research goal, it can be used as a membrane-free compartment for isolated biochemical reactions mimicking specific cell functions or as a template for the creation of lipid bilayers.
More complex designs allow the encapsulation of droplets inside larger droplets to mimic the compartmentalization of natural cells and to provide better timely control of the reactions since the reagents are not all mixed in the dispersed phase before droplet formation. Majumder et al., 2019 [17] recently reviewed how droplets can generate artificial cells.
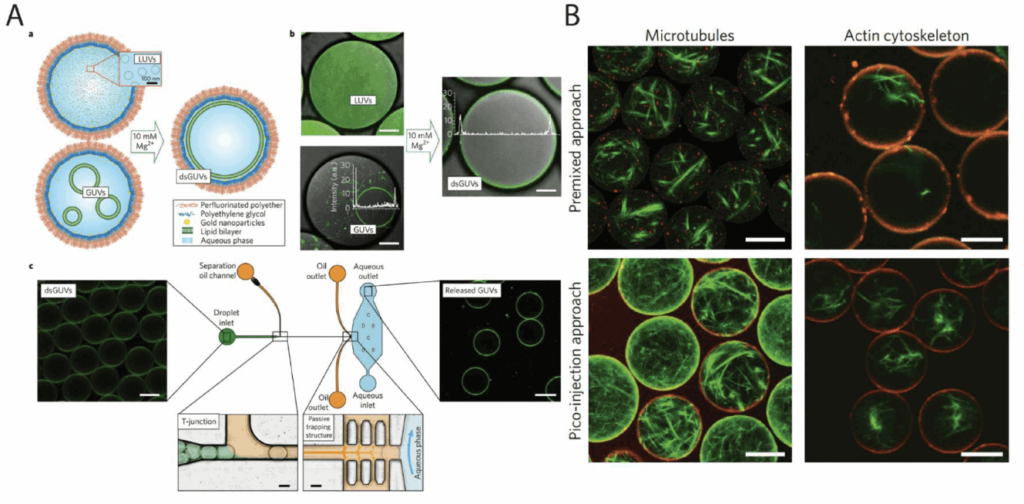
Key takeaways from droplet encapsulation
Droplet encapsulation provides the ability to create microreactors in a highly controlled manner and in large quantities in the form of monodispersed droplets, which affords clear advantages to applications in biological research. Also, microfluidics setups are flexible and can be adjusted to fit several applications, which explains their rapid adoption outside the physics and fluidics fields.
For researchers who do not have a microfluidics background, the task of setting up the first microfluidics experiment might seem daunting. However, several solutions are aimed at turning microfluidics into an easy-to-use tool that can be employed without previous expertise. For example, an easy droplet-generator pack, and an automatic droplet size regulator pack are available for researchers interested in including droplets in their current research without learning about an entirely new field.
Review done thanks to the support of the Protomet H2020-MSCA-ITN-2018-Action “Innovative Training Networks”, Grant agreement number: 813873
Author: Camila Betterelli Giuliano, PhD
Partnership[at]microfluidic.fr
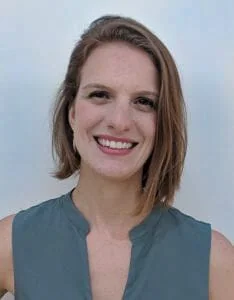
References
- N. Wen, Z. Zhao, B. Fan, D. Chen, D. Men, J. Wang and J. Chen. Development of Droplet Microfluidics Enabling High-Throughput Single-Cell Analysis. Molecules, vol. 21, 7, 2016.
- T. Schneider, J. Kreutz and D. T. Chiu. The Potential Impact of Droplet Microfluidics in Biology. Analytical Chemistry, vol. 85, 7, 2013.
- Zubaite, G., Simutis, K., Galinis, R., Milkus, V., Kiseliovas, V., & Mazutis, L. Droplet Microfluidics Approach for Single-DNA Molecule Amplification and Condensation into DNA-Magnesium-Pyrophosphate Particles. Micromachines, 8(2), 62, 2017.
- L. Shang, Y. Cheng, and Y. Zhao. Emerging Droplet Microfluidic. Chemical Reviews, vol. 117, 2017.
- T. S. Kaminski, O. Scheler and P. Garstecki. Droplet microfluidics for microbiology: techniques, applications and challenges. Lab on a Chip, vol. 16, 2016.
- B. B. Wang, X. D. Wang, T. H. Wang and W.M. Yan. Electrocoalescence behavior of two identical droplets with various droplet radii. Applied Thermal Engineering, vol 111, 25, 2017.
- M.Sesen, T. Alan and A. Neild. Microfluidic on-demand droplet merging using surface acoustic waves. Lab on a Chip, vol. 14, 2014.
- J. Sivasamy, Y. C. Chim, T. N. Wong, N.T. Nguyen and L. Yobas. Reliable addition of reagents into microfluidic droplets. Microfluidics and Nanofluidics, vol. 8, 3, 2010.
- H. Yuan, Y. Pan, J. Tian, Y. Chao, J. Li, H. C. Shum. Electricity-free picoinjection assisted droplet microfluidics. Sensors and Actuators B: Chemical, vol 298, 2019.
- A. R. Abate, T. Hung, P. Mary, J. J. Agresti and D. A. Weitz. High-throughput injection with microfluidics using picoinjectors. PNAS, 107 (45), 2010.
- F. W. Y. Chiu and S. Stavrakis. High‐throughput droplet‐based microfluidics for directed evolution of enzymes. Electrophoresis, 2019
- L. D. van Vliet and F. Hollfelder. Microfluidic Droplets and Their Applications: Diagnosis, Drug Screening and the Discovery of Therapeutic Enzymes. IFMBE Proceedings, 2019.
- P. Shahi, S. C. Kim, J. R. Haliburton, Z. J. Gartner & Adam R. Abate. Abseq: Ultrahigh-throughput single cell protein profiling with droplet microfluidic barcoding. Nature Scientific Reports, 7, 2017.
- E. Z. Macosko, A. Basu, R. Satija, J. Nemesh, K. Shekhar, M. Goldman, I. Tirosh, A. R. Bialas, N. Kamitaki, E. M. Martersteck, J. J. Trombetta, D. A. Weitz, J. R. Sanes, A. K. Shalek, A. Regev and S. A. McCarroll. Highly Parallel Genome-wide Expression Profiling of Individual Cells Using Nanoliter Droplets, Cell, vol. 161, 5, 2015.
- S. L. Sjostrom, Y. Bai, M. Huang, Z. Liu, J. Nielsen, H. N. Joenssona and H. A. Svahn. High-throughput screening for industrial enzyme production hosts by droplet microfluidics. Lab on a chip, issue 4, 2014.
- H. Feng, T. Zheng, M. Li, J. Wu, H. Ji, J. Zhang, W. Zhao and J. Guo. Droplet-based microfluidics systems in biomedical applications. Electrophoresis, vol. 40, 11, 2019.
- S. Majumder, N. Wubshet and A. P. Liu. Encapsulation of complex solutions using droplet microfluidics towards the synthesis of artificial cells. Journal of Micromechanics and Microengineering, vol. 29, 8, 2019.
- Weiss, M., Frohnmayer, J., Benk, L. et al. Sequential bottom-up assembly of mechanically stabilized synthetic cells by microfluidics. Nature Mater 17, 89–96 (2018)